
Tolterodine is chemically known as (R)-N,N-disiopropyl-3-(2-hydroxy-5-methyl phenyl)-3-phenyl propyl amine. Tolterodine acts as a muscarinic receptor antagonist. It is useful in the treatment of urinary incontinence [1]. Tolterodine tartrate acts by relaxing the smooth muscle tissues in the walls of the bladder by blocking cholinergic receptors[2]. Tolterodine tartrate [3] is marketed by Pharmacia & Upjohn in the brand name of Destrol®.
The present invention relates to a novel process for the preparation of N,N-diisopropyl-3-(2-hydroxy-5-methylphenyl)-3-phenylpropanamide (4); a key intermediate for the preparation of Tolterodine (1). Some different approaches have been published [4-8] for the preparation of N,N-diisopropyl-3-(2-hydroxy-5-methylphenyl)-3-phenylpropanamide (4). These methods involve multistep synthesis using hazardous, expensive reagents and some of the methods [6] involve activators like Grignard reagents, LDA, n-butyl lithium, Lewis acids. Hence there is a need to develop an alternative, plant friendly procedure for the preparation of N,N-diisopropyl-3-(2-hydroxy-5-methylphenyl)-3-phenylpropanamide (4) from 3,4-dihydro-6-methyl-4-phenylcoumarin (2) (Fig1).
Tolterodine (1), Methyl 3-(2-hydroxy-5-methylphenyl)-3-phenylpropanoate (3) and N,N-diisopropyl-3-(2-hydroxy-5-methylphenyl)-3-phenylpropanamide (4).
Improved
one-pot synthesis of N,
N-diisopropyl-3-(2-Hydroxy-5-methylphenyl)-3-phenyl propanamide; a key
intermediate for the preparation of racemic Tolterodine
Ring opening reactions of dihydrocoumarins are well known in literature[9-11]. But in the present invention, we have described a new methodology (Scheme 1 & Scheme2) for the preparation ofN,N-diisopropyl-3-(2-hydroxy-5-methylphenyl)-3-phenylpropanamide (4) by using inexpensive and commercially vailable starting materials like 3, 4-dihydro-6-methyl 4-phenylcoumarin (2), which was synthesized from p-cresol and trans-cinnamic acid [12].

Scheme 1
N,N-diisopropyl-3-(2-hydroxy-5-methylphenyl)-3-phenylpropanamide 4.

Scheme 2
N-Isopropyl-3-(2-hydroxy-5-methylphenyl)-3-phenylpropanamide 5.
3,4-Dihyhydro-6-methyl 4-phenylcoumarin (2) reacts with diisopropylamine (6) in presence of acetic acid gives N,N-diisopropyl-3-(2-hydroxy-5-methylphenyl)-3-phenylpropanamide (4) at room temperature. This process of compound 4 is very useful for commercialization of Tolterodine 1 in plant.
Scheme 1
N,N-diisopropyl-3-(2-hydroxy-5-methylphenyl)-3-phenylpropanamide 4.
Scheme 2
N-Isopropyl-3-(2-hydroxy-5-methylphenyl)-3-phenylpropanamide 5.
General procedure for the synthesis of compounds 4-4c & 5-5c
To a solution of 3,4-dihyhydro-6-methyl 4-phenylcoumarin 2 (10 g, 42 mmol) in diisopropylether (200 mL), N,N-diisopropylamine (33.95 g, 336 mmol) and acetic acid (10 g, 168 mmol) were added at room temperature. The suspension was stirred for 16 h at room temperature. The reaction mass was concentrated, the resulting residue was crystallized with D.M.Water (50 mL) and diisopropyl ether (50 mL) mixture to gave N,N-diisopropyl-3-(2-hydroxy-5-methylphenyl)-3-phenylpropanamide 4 (10.6 g, 75% yield).N,N-diisopropyl-3-(2-hydroxy-5-methylphenyl)-3-phenylpropanamide 4
IR (KBr) cm-1: 3024 (Aromatic C-H, str.), 2949, 2904, 2869 (Aliphatic C-H, str.), 1630 (C═O, str.), 1609, 1555, 1510 (C═C, str.), 1469, 1459 (CH2 bending), 1270 (C-N, str.), 1072 (C-O, str.), 788, 769 (Aromatic CH Out-of-plane bend). 1H NMR (300 MHz, DMSO-d6) δ 1.04 (d, 12H), 2.089 (s, 3H), 2.79 (m, 2H), 3.037 (m, 2H), 4.702 (t, 1H), 6.6 (d, 1H), 6.75 (d, 2H), 7.127-7.246 (m, 5H). 13C NMR (125 MHz, DMSO-d6) δ 19.39, 20.36, 45.69, 115.33, 125.70, 127.20, 128.15, 130.60, 144.43, 152.23, 173.37. MS m/z: 340 [(M + H)+].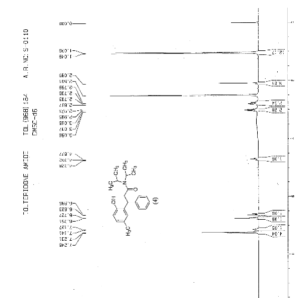
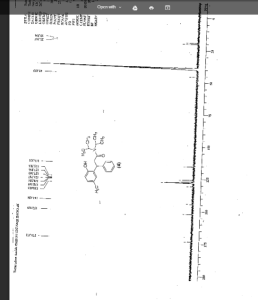
Improved one-pot synthesis of N, N-diisopropyl-3-(2-Hydroxy-5-methylphenyl)-3-phenyl propanamide; a key intermediate for the preparation of racemic Tolterodine
2Engineering Chemistry Department, AU college of Engineering, Andhra University, Visakhapatnam 530003, Andhra Pradesh, India
The electronic version of this article is the complete one and can be found online at:http://www.sustainablechemicalprocesses.com/content/2/1/2
http://www.sustainablechemicalprocesses.com/content/2/1/2/additional

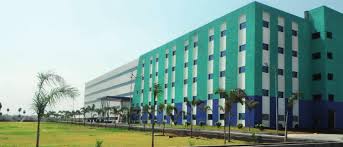
http://www.sustainablechemicalprocesses.com/content/2/1/2/additional

Srinivas garaga
scientist at Aurobindo Pharma
Chemical Research and Development Department, Aurobindo Pharma Ltd
/////